What actually goes on inside a cyclist's body during sprinting, sustained and endurance efforts?
Your body generates cycling energy through multiple different systems. But what are they, how do they interact, and how can you turbo-charge all of them?
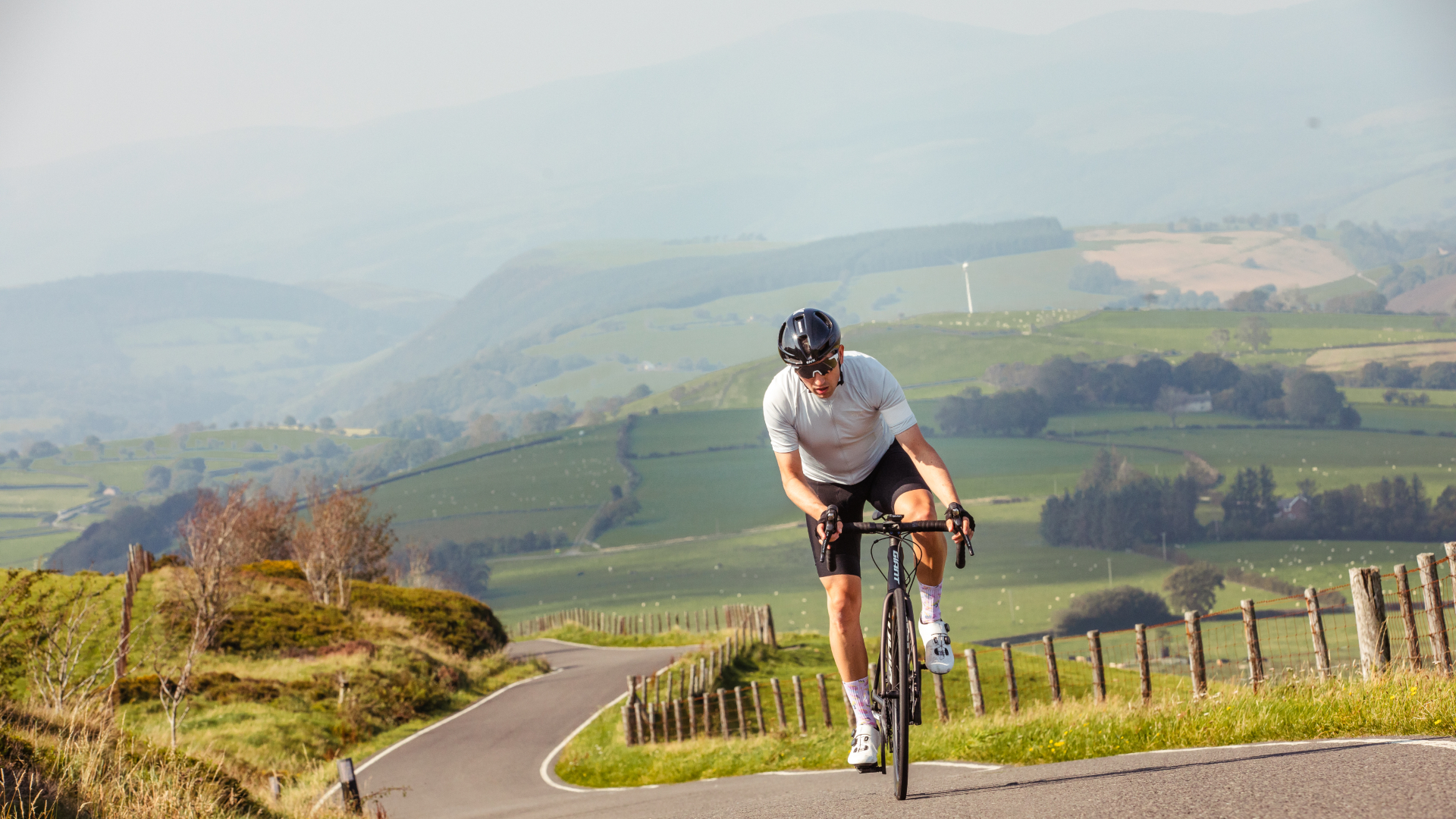
Have you ever wondered how does your body power through intense cycling workouts? We’re going to take an in-depth look at a cyclist's body’s energy systems – the processes going on in all of us every time we ride.
ATP: the master molecule for cycling performance
Now, this might be a little geeky from the get-go but it'll all become clear – we promise!
When a cyclist sprints, the mechanism at play is called 'ATP resynthesis', which involves the reproduction of ATP - the cellular energy currency - from the use of ADP, organic phosphate (Pi), and other substrates including phosphocreatine, carbohydrates, and fats.
Cells don’t tolerate a high level of ADP and Pi very well and that’s why the body must work tirelessly to resynthesise the ATP which drives our muscles – essentially, cyclists' bodies are continually playing catch up this energy molecule.
There are multiple ways the body can go about resynthesising ATP and they occur all at the same time – but don't worry, we're going to go through it all one energy system at time. Let's jump in!
Oh, and for the curious, ATP stands for adenosinetriphosphate and ADP is adenosinediphosphate.
1. What happens inside cyclists' bodies when sprinting?
AKA: Any time you try to outsprint your mate to a road sign
Get The Leadout Newsletter
The latest race content, interviews, features, reviews and expert buying guides, direct to your inbox!
Technical name: Phosphocreatine system (PCr)
Cycling purpose: For 10-15 seconds at max effort
What is it?
The phosphocreatine system uses the phosphocreatine stored in the muscles to put a molecule of phosphate back on ADP and produce ATP. “Breaking phosphocreatine to resynthesise ATP is a very quick way of breaking something down to build something else back up: the same amount of energy released by the breakdown of PCr is used to resynthesise ATP,” says Mark Burnley, senior lecturer of sports and exercise physiology at the University of Kent.
So why don’t we keep a lot of ATP in the muscles and use that, instead of storing phosphocreatine to create new ATP?
"ATP is quite a heavy molecule,” says Burnley. “You don’t really want a lot of it about. In fact, if you had to store enough ATP to run a marathon, you would have to increase your body mass by about 100kg. And that’s why we also have fat and carbohydrates stored as energy [read more below], because they’re relatively light compared to ATP.”
Why it matters
The PCr system can re-form ATP very quickly and does not need oxygen to perform this task, and the system does not generate byproducts. However, because the phosphocreatine stores in the muscles are limited, the body uses the other energy systems to supply its longer-duration energy demands. That’s why you can’t sprint for a very long time, and why you need to use different energy pathways to produce ATP to ride for a long time. While sprinting, the body is already preparing for subsequent efforts using different mechanisms.
“As soon as you break down phosphocreatine, you produce creatine and inorganic phosphate,” says Burnley. “And that immediately signals to the mitochondria to start increasing oxygen consumption. As far as we can tell, the breakdown of phosphocreatine controls the aerobic metabolism too.”
In a nutshell, the PCr system does not only produce ATP quickly for immediate use. It also preempts what happens next, when the other systems kick in and take over the majority of ATP production. The system is active and has an impact from the start of a ride, every time you sprint, even after hours in the saddle.
Myths and misunderstandings
The PCr system does not work on its own. It is always active in conjunction with the other systems. Even for longer efforts above threshold, the PCr system is involved in producing ATP.
Put it to use
The best way to train this energy system is to do sprint training. If you’re trying to improve your peak power, you need to have longer rests in between sprints. If, on the other hand, you use sprints to improve aerobic performance, your sprint sessions should involve shorter recoveries. These sprints will also train and activate the endurance pathways, which the body requires to perform longer efforts at lower intensities. Sprint training, paradoxically, is an excellent aerobic stimulus and won't necessarily lead to cyclists' getting big legs and the heavy-set cycling physique of a track sprinter.
2. What happens inside cyclists' bodies during sustained hard efforts?
AKA: A rider trying to break away from the bunch
Technical name: Glycolytic system/glycolysis or ‘anaerobic’
Cycling purpose: Max effort of 90-120 seconds or 3-8min sub-max, e.g. attacking to get away.
What is it?
Instead of using phosphocreatine, the glycolytic system relies on glucose or glycogen (glucose stored in the muscles and liver) to resynthesise ATP. Although the process can happen without oxygen – and it’s often called anaerobic – it’s also seen as the first stage of the aerobic system. The system is good at producing a lot of energy relatively quickly, but it can do so only for a short period because too many byproducts build up too quickly. Glycolysis produces energy about 100 times faster than the aerobic system.
Once again, there is no time or threshold when one system stops working and another kicks in. “The systems never work in isolation, but always work together,” says cycling coach and exercise physiologist James Spragg. “There’s always some aerobic, glycolytic, and phosphocreatine system working. Just their percentages differ, and also where that energy is coming from.”
Why it matters
The glycolytic system takes a glucose molecule (either from the bloodstream or glycogen) and breaks it down into pyruvate. This is why it’s important that you're fuelling optimally, with carbohydrates before and during intense rides: you don’t want to run out of glycogen or glucose.
At this point, the body has a decision to make: either it can send pyruvate through the aerobic metabolism system (called the Krebs cycle) or, failing that, it has to convert pyruvate into lactate. Counter to common misconception, lactate is not a waste product; it’s another type of fuel.
Myths and misunderstandings
“We know the glycolytic system produces lactate, which is the correct name in use – different from lactic acid, which is found in milk,” says Spragg. “Lactate has no role in cellular muscle fatigue.”
Our muscle cells can use lactate as a fuel or use it to produce ATP. The traditional notion of lactate being a ‘bad guy’ and causing ‘lactic burn’ in the muscles is a myth.
“You can almost think of the lactate system as a buffer,” explains Spragg. “There is even some suggestion that some of the increase in blood lactate when we start exercising is actually non-working muscles producing lactate to be shipped to the working muscles as a fuel. It’s called the lactate shuttle hypothesis.”
Another myth is the presumed mutual exclusivity between the anaerobic system (chemical reactions without oxygen), the aerobic system (with oxygen), and the substrates they use. There is always crossover. For example, lactate is produced both anaerobically and aerobically.
Put it to use
Any effort of 30 to 90 seconds relies mostly on glycolysis; for example, the 1km time trial or when a rider tries to break off the bunch by means of a hard, sustained effort. To train this system, you need to work at high intensities (above 90 per cent of VO2max) for short periods of time, or at around lactate threshold for longer intervals. Most coaches recommend polarised cycling training, with 80 per cent of training sessions performed at low intensity, and only 20 per cent above lactate threshold.
3. What happens inside cyclists' bodies during endurance efforts?
AKA: Any long ride
Technical name: Oxidative phosphorylation or ‘aerobic’
Cycling purpose: Any effort performed at a lower intensity and/or longer duration
What is it?
Once the body has used phosphocreatine, glucose, and lactate to resynthesise ATP, it can still take a different path to provide energy to the working muscles. That happens when the exercise duration is longer and the intensity lower. In this case, enzymes inside the mitochondria oxidise nutrients (fatty acids, but also glucose and lactate). Think of it as the motor diesel that allows you to go long – though there’s a high cost to it.
“The oxidative system requires so much oxygen, more than we can get onboard quick enough to meet demand at high intensities,” says Nicholas Willsmer, lecturer in sports performance at the University of Bath. “And despite the fact that it can produce 20 times more energy [than glycolysis], its turnover of ATP is slower. So the intensity of the exercise has to come down.”
When you ride for a long period, and at a lower intensity, this is the main energy system your body uses.
Why it matters
Although it requires a lot of oxygen to perform its task and can’t sustain high intensities for an extended time, oxidative phosphorylation is the preferred system for any prolonged activity at low power. This training is the classic ‘baking of the cake’ that builds your cycling endurance.
Myths and misunderstandings
The principal myth about this and other energy systems is that they work independently. Rather, all of them are always in play but the proportion that each contributes to energy generation changes according to the intensity of the exercise. Furthermore, it’s not only fat that is oxidised through phosphorylation. The body can use glucose (if preceded by glycolysis) or proteins – although the latter is counterproductive for sports performance, as it requires the breaking down of muscle.
Put it to use
The best way to train this system is to ride long distances at an easy pace, below 75 per cent of your max. At low intensity, this system is working at its maximum rate of ATP turnover. Recent studies have shown that the amount of power you can sustain within this cycling training zone is connected to how many contact points there are between capillaries and slow-twitch muscle fibres.
The more capillaries there are in the body, the more oxygen is transported from the lungs to the muscles and, consequently, the more oxygen can get into the cells. The long and slow rides are meant to develop central adaptations, such as growing more capillaries, raising cardiac output and oxygen levels in the blood.
If you don't understand some of the fitness terms used check out our A to Z of training jargon.
Thank you for reading 20 articles this month* Join now for unlimited access
Enjoy your first month for just £1 / $1 / €1
*Read 5 free articles per month without a subscription
Join now for unlimited access
Try first month for just £1 / $1 / €1
Nick Busca is a freelance cycling and triathlon journalist. He is also a certified triathlon coach and personal trainer.